Narcosis Diving
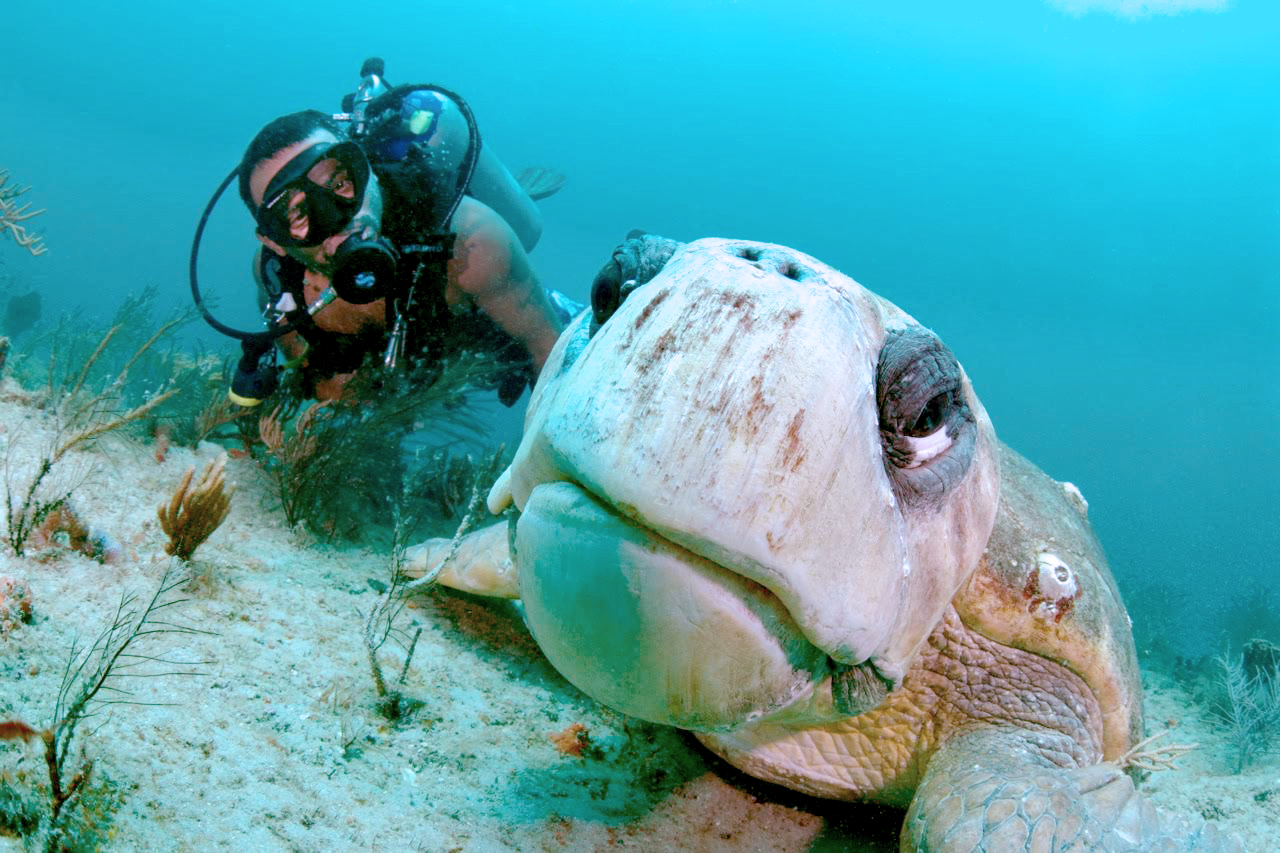
Mammals are not ideal diving machines
Mammals, as a rule, are warm blooded air breathers, and that is not ideal for the conditions of the deep sea. Air-filled lungs are easily crushed by the pressure of a hundred meters or more, squeezing out any oxygen they held. Water has a high specific heat and anyone who has taken “a polar plunge” for charity knows how quickly it can remove heat from a warm blooded animal. Yet, marine mammals have thrived in the ocean, and have evolved adaptations that allow them to dive to extraordinary depths. Here is how they do it.
Narcosis, also called 'decompression sickness' is a natural phenomenon due to excess nitrogen that consequently acts on the nervous system and causes strange behaviour. Actually, when diving, we breath in a mixture of several gases and the pressure when diving is going to have a direct impact on them.
The problems with going deep
Pressure increases by one atmosphere for every ten meters that you descend into the ocean. At sea level we experience 14.7 pounds of pressure on every square inch of our bodies. However, sea water is a lot denser and the further you go down, the greater the pressure gets. Pressure, doesn’t affect fluid filled tissues, but it immediately reduces the volume of the air filled spaces. You and I can feel pressure in the air-filled sinuses of our face as we dive to the bottom of a pool (about three meters.) A diving marine mammal will have all of the air squeezed out of its lungs within a few dozen meters. Pressure also increases the rate at which gases dissolve into the liquid portions of our blood. If a diving marine mammal tries to return to the surface rising too quickly, those dissolved gases can form bubbles that lodge in blood vessels of critical organs, creating a condition called decompression sickness or “the bends.” Early deep sea divers called this condition the bends because of the intense joint pain it could cause. The bends can be debilitating and deadly not just in people, but in marine mammals as well. Click to read more about the bends.
Oxygen consumption can become an issue for you and I in less than a minute if deprived of new oxygen. Try squeezing your nose and holding your breath – how long can you last? The best free divers in the world can’t hold their breath for longer than five minutes, yet some marine mammals can stay down without a breath for as long as ninety minutes.
Nitrogen narcosis. Air is 70% nitrogen, but under normal atmospheric conditions almost none of it gets dissolved in our blood. That’s a good thing, because when it does, it can cause a drunken-like condition called nitrogen narcosis. Under higher pressures, greater levels of nitrogen can dissolve into a diving animals blood and eventually becomes toxic. It can lead to impaired judgement and eventually death. Nitrogen narcosis typically limits air breathing scuba divers to depths of one hundred feet or less. Anyone human diving beyond that typically uses a gas mixture with less nitrogen.
The ocean is cold. Even in the tropics, below 200 meters ocean water temperatures approach freezing. Warm blooded mammals need to maintain a constant body temperature to survive. In addition, water’s high heat capacity can remove heat from the body nearly twenty times faster than air.
Mammalian bodies float. Ever tried to swim to the bottom of a swimming pool? It takes a lot of work. That’s because the air and fat in most mammals tissue cause us to float.
William Beebe and Otis Barber in the Bathysphere. This first true deep diving submersible was little more than a steel ball attached to a heavy line, image from Wikipedia. Osu okc.
How humans adapt to these problems
- Pressure: Pressure normally limits scuba divers to less than thirty meters. Submarines use closed steel and titanium spherically shaped containers with small thick windows to withstand increasing pressure.
- Oxygen: Scuba divers use compressed air, or other gas mixtures stored in steal containers.
- Nitrogen narcosis and decompression sickness: Scuba divers follow strict diving charts with slow assents and descents. Divers who experience nitrogen narcosis or the decompression sickness may have to spend time in specialized decompression chambers.
- The Cold: Scuba divers wear thick neoprene wet suits or insulated dry suits.
- Buoyancy: Scuba divers wear lead weights on their belts and submersibles may have thousands of pounds of lead weights attached to the sub to help them sink.
Marine Mammal Adaptations
The sperm whale, seen in the diagram above, is the diving champion of marine mammals. Sperm whales can stay under water for over 90 minutes and dive to depths of nearly 10,000 ft. It has an extraordinary array of adaptations that allow it to dive so deep. All marine mammals can make dives that are deep compared to human beings. Here is how they do it.
Elephant seals dive to extraordinary depths. Image from Wikipedia
Problem 1: Pressure
If you dive to the bottom of a swimming pool, the first thing you might notice is that your ears hurt. This is because of the difference in pressure between the air in your ears and the water. Air (as a gas) can be compressed, while water (as a liquid) cannot. At sea level a body experiences 14.7 pounds per square inch of pressure (1 atmosphere). For a diving marine mammal, another atmosphere of pressure is added every ten meters that they go down. Thus, a male elephant seal diving to 1,000 meters is experiencing 100 times the pressure that he would at the surface.
Over evolutionary time, most marine mammals have lost their external ears and sinuses. Without air-filled ears, a diving marine mammal does not suffer the effects of changing pressure. Sea lions and fur seals do have ears. During a dive their ears will fill with a bloody fluid, forcing any air out.
Human beings store oxygen primarily in their lungs than any other place. In preparation to hold your breath, it would make sense that you would take a deep breath first. For diving marine mammals, this presents two problems. First, air is buoyant, making diving difficult. Second, as was mentioned above, air is easily compressed, leading to a potential collapse of the lungs. Most marine mammals have lungs that are able to collapse and re-inflate easily. They tend to be long and tubular with built-in protective rings to keep valves open. Also, marine mammals tend to exhale right before a dive. They have very muscular and efficient lungs which can exhale up to 90% of the air in their lungs in any give breath (an athletic human can do around 10%.) Thus, by removing the air from their body, a diving marine mammal has very little problems with changing pressure. No air, no problem.
The heart rate of a diving Weddell Seal. Image from Alaska Sea Grant, used with permission
Problem 2: Oxygen Storage
So if marine mammals exhale before they dive, how do their muscles get the oxygen they need to work? The answer is that they store oxygen in their blood, and in their muscles rather than in their lungs. Marine mammals have a very high blood to body volume ratio. Marine mammals also have a higher percentage of red blood cells than most mammals (human = 36%, seals = 50%.) By comparison, this makes their blood very thick and viscous but much more adept at oxygen storage. Marine mammals also have a high concentration of hemoglobin in their blood and myoglobin in their muscles. Both of these molecules have a high affinity for oxygen atoms and are used to store oxygen in body tissue. Click the link to check out how human free diving champions match up against marine mammals for deep sea dives.
The mammalian diving reflex allows mammals to lower their heart rate and ultimately survive submersion in water for extended periods of time. Bradycardia, as it is also known, is triggered by cold water contact to the nerves of the face. It occurs in all mammals, but to a much greater extent in marine mammals. Weddell seals have been measured to lower their heart rate to as low as four beats per minute when submerged in cold water.
When exercising, we say that when humans have run out of oxygen they have gone anaerobic. This means that muscle cells do not have sufficient oxygen to break sugar apart through aerobic respiration. This is very taxing on muscles and leads to soreness and fatigue. In marine mammals, most body organs appear to switch to anaerobic respiration while diving without suffering the same ill effects. We still don’t know exactly how they do this.
A Weddell seal is shown wearing a Sea Mammal Research Unit Conductivity Temperature Depth tag in McMurdo Sound Antarctic. Photograph by Dan Costa. Image from Actionbioscience.org
Problem 3: Decompression Sickness and nitrogen narcosis
Marine mammals regularly make deep dives and return to the surface very quickly. So why don’t they have problems with “the bends?” It’s simple – they exhale before they dive. No air means no problem. In addition, many marine mammals have an extensive “net” of blood vessels feeding into their brain. It’s known as the “retia mirabilia,” and it likely serves several functions, but one of them is capturing bubbles that may form in the blood stream. “Until recently the dogma was that marine mammals have anatomical and physiological and behavioral adaptations to make the bends not a problem,” said MMC Director Michael Moore. “There is no evidence that marine mammals get the bends routinely, but a look at the most recent studies suggest that they are actively avoiding rather than simply not having issues with decompression.” – Woods Hole Oceanographic Institute.
Problem 4: The Cold
Many human scuba divers have known the pain and discomfort associate with having a small hole in their wetsuit during a cold water dive. Water dissipates heat from the body much faster than air. A person who falls in water near the freezing point will be hypothermic within a few minutes, yet marine mammals dive to depths where the temperatures approach freezing.
The most obvious way that marine mammals stay warm is that they tend to be large and rather “sausage shaped.” This shape gives them a low surface area to volume ratio. Per unit volume, there is less skin exposed to cold moving water. Marine mammals also have a lot of blood relative to their body size. Water has a high heat capacity and does a nice job of maintaining body temperature. Most marine mammals have a thick layer of fat know as “blubber.” This fat layer also serves as calorie storage for marine mammals that undergo long periods of fasting. Smaller marine mammals tend to have highly insulated layers of fur. The extreme example of this is the sea otter. Sea otter fur is two layers thick, and very effective at trapping air to aide in insulation. Sea otters may have as many as one million hairs per square inch. That’s ten times as many hairs as the average human has on their whole head.
ARKive Image. Bowhead Whale Caught By Subsistance Hunters. (these whales have as much as two feet of insulating blubber)
Problem 5: Buoyancy
No part of a mammal is more buoyant than the air in their lungs. For marine mammals, the key to reducing this buoyancy is to exhale before diving. Removing oxygen from the lungs makes animals slightly negatively buoyant. In order to save energy diving, marine mammals will often sink in a “sleep-like state” during the descent of a long dive. This is only possible after the lungs have been collapsed.
Marine diving champions
- thick billed murres – weighing only a pound or two, they can dive up to 100 meters
- elephant seals – to 1500 meters, over 60 minutes, spend 90% of time at sea diving
- emperor penguins – up to 500 meters
- leatherback sea turtles – up to 1200 meters
- sperm whales – up to 2032 meters, regularly staying down for 90 minutes
- Cuvier’s beaked whales – up to 2992 meters, stayed underwater 138 minutes
- to read more go to BBC: Extreme Divers Defy Explanation
Questions for Research
- The very fact that “marine” mammals exist is an extraordinary example of evolution. To illustrate this, describe some of the physiological difficulties of being the super hero Aquaman.
- Describe the recently evolved adaptation that has allowed the The Bajau people to become better divers. Be specific to how the adaptation is helpful.
- How do marine mammals deal with the extreme pressure they encounter on their dives? If the text above isn’t enough, check out this great article from Scientific American.
- How do marine mammals deal with oxygen storage? It may help you to learn what scientists have recently discovered about the myoglobin found in Marine Mammals.
- What kinds of things have human free dive champions learned that have greatly extended their dives? Based on what you have read and heard so far about marine mammals, are their any overlaps in behavior between marine mammals and human free divers?
- How do marine mammals avoid freezing to death while diving?
- Examine the attached diagram of a typical Fin Whale feeding dive. Look at the fluking pattern (these are beats of their tail). How hard are they swimming when they dive? How hard are they swimming when they return to the surface?
- We have recently learned that diving whales can get decompression sickness (The Bends.) What have we learned, and how might the US navy be putting marine mammals in harms way?
- Read the description of the sperm whale , and explain how the spermaceti organ can help to regulate buoyancy in sperm whales.
This figure shows the diving behavior of a male elephant seal. Dives lasting 20-40 minutes of nearly vertical profiles are followed by very short breaks. Image from, National Marine Mammal Laboratory, Alaska Fisheries Science Center, NOAA Fisheries, used with permission.
A is used to treat some diving disorders and for training divers to recognise the symptoms.are specifically arising from. The and of these may present during a dive, on surfacing, or up to several hours after a dive. Have to breathe a gas which is at the same pressure as their surroundings , which can be much greater than on the surface. The ambient pressure underwater increases by 1 (100 kPa) for every 10 metres (33 ft) of depth.The principal conditions are: (which covers and );;;; and (burst lung). Although some of these may occur in other settings, they are of particular concern during diving activities.The disorders are caused by at the high pressures encountered at depth, and divers will often breathe a gas mixture different from air to mitigate these effects., which contains more and less, is commonly used as a breathing gas to reduce the risk of decompression sickness at (up to about 40 metres (130 ft)).
May be added to reduce the amount of nitrogen and oxygen in the gas mixture when diving deeper, to reduce the effects of narcosis and to avoid the risk of oxygen toxicity. This is complicated at depths beyond about 150 metres (500 ft), because a helium–oxygen mixture then causes high pressure nervous syndrome. More exotic mixtures such as, a hydrogen–helium–oxygen mixture, are used at extreme depths to counteract this.
The recompression chamber at the used for treating DCS and training(DCS) occurs when gas, which has been breathed under high pressure and dissolved into the, forms bubbles as the pressure is reduced on ascent from a dive. The results may range from pain in the joints where the bubbles form to blockage of an leading to damage to the, or death.
While bubbles can form anywhere in the body, DCS is most frequently observed in the shoulders, elbows, knees, and ankles. Joint pain occurs in about 90% of DCS cases reported to the, with symptoms and skin manifestations each present in 10% to 15% of cases. DCS is very rare in divers.
The table below classifies the effects by affected organ and bubble location. The pulmonary circulationIf the compressed air in a diver's cannot freely escape during an ascent, particularly a rapid one, then the lung tissues may rupture, causing (PBT).
The air may then enter the producing (AGE), with effects similar to severe. Although AGE may occur as a result of other causes, it is most often secondary to PBT. AGE is the second most common cause of death while diving ( being the most common stated cause of death). Gas bubbles within the arterial circulation can block the supply of blood to any part of the body, including the brain, and can therefore manifest a vast variety of symptoms. The following table presents those signs and symptoms which have been observed in more than ten percent of cases diagnosed as AGE, with approximate estimates of frequency.Other conditions that can be caused by pulmonary barotrauma include,.
Narcosis can produce, making it difficult to read multiple gauges.is caused by the pressure of dissolved gas in the body and produces to the. This results in alteration to thought processes and a decrease in the diver's ability to make judgements or calculations. It can also decrease, and worsen performance in tasks requiring. As depth increases, so does the pressure and hence the severity of the narcosis.
The effects may vary widely from individual to individual, and from day to day for the same diver. Because of the perception-altering effects of narcosis, a diver may not be aware of the symptoms, but studies have shown that impairment occurs nevertheless. Since the choice of breathing gas also affects the depth at which narcosis occurs, the table below represents typical manifestations when breathing air.
An EEG recording netis the least of all gases, and divers may use containing a proportion of helium for dives exceeding about 40 metres (130 ft) deep. In the 1960s it was expected that helium narcosis would begin to become apparent at depths of 300 metres (1,000 ft).
However, it was found that different symptoms, such as, occurred at shallower depths around 150 metres (500 ft). This became known as, and its effects are found to result from both the absolute depth and the speed of descent. Although the effects vary from person to person, they are stable and reproducible for each individual; the list below summarises the symptoms observed underwater and in studies using simulated dives in the dry, using and (EEG) monitors. Signs and symptoms of HPNSSymptomNotesImpairmentBoth intellectual and are. A 20% decrease in the ability to perform calculations and in is observed at 180 metres (600 ft), rising to 40% at depths of 240 metres (800 ft)Dizziness, and may occur in divers at depths of 180 metres (600 ft). Animal studies under more extreme conditions have produced.Tremorsof the hands, arms and torso are observed from 130 metres (400 ft) onward. The tremors occur with a frequency in the range of 5–8 (Hz), and their severity is related to the speed of compression; the tremors reduce and may disappear when the pressure has stabilised.EEG changesAt depths exceeding 300 metres (1,000 ft), changes in the (EEG) are observed; the appearance of (4–6 Hz) and depression of (8–13 Hz).SomnolenceAt depths beyond the onset of EEG changes, test subjects intermittently, with 1 and 2 observed in the EEG.
Even when decompressed to shallower depths, the effect continues for 10–12 hours.Oxygen toxicity. During Professor Kenneth Donald carried out extensive testing for oxygen toxicity in divers. The chamber is pressurised with air to 3.7 bars (370 kPa; 54 psi). The subject in the centre is breathing 100% oxygen from a mask.Although is essential to life, in concentrations greater than normal it becomes, overcoming the body's natural defences , and causing in any part of the body.
The and are particularly affected by high of oxygen, such as are encountered in diving. The body can tolerate partial pressures of oxygen around 0.5 (50 kPa; 7.3 psi) indefinitely, and up to 1.4 bars (140 kPa; 20 psi) for many hours, but higher partial pressures rapidly increase the chance of the most dangerous effect of, a resembling an. To oxygen toxicity varies dramatically from person to person, and to a much smaller extent from day to day for the same diver. Prior to convulsion, several symptoms may be present – most distinctly that of an.During 1942 and 1943, Professor Kenneth W Donald, working at the Admiralty Experimental Diving Unit, carried out over 2,000 experiments on divers to examine the effects of oxygen toxicity. To date, no comparable series of studies has been performed. In one seminal experiment, Donald exposed 36 healthy divers to 3.7 bars (370 kPa; 54 psi) of oxygen in a chamber, equivalent to breathing pure oxygen at a depth of 27 metres (90 ft), and recorded the time of onset of various signs and symptoms. Five of the subjects convulsed, and the others recovered when returned to normal pressure following the appearance of acute symptoms.
The table below summarises the results for the relative frequency of the symptoms, and the earliest and latest time of onset, as observed by Donald. The wide variety of symptoms and large variability of onset between individuals typical of oxygen toxicity are clearly illustrated. Signs and symptoms of oxygen toxicity observed in 36 subjectsSigns and symptomsFrequencyEarliest onset (minutes)Latest onset (minutes)19911515predominanceNote.
^ Brubakk, Alf O; Neuman, Tom S, eds. '9: Pressure Effects'. Bennett and Elliott's physiology and medicine of diving (5th Revised ed.). United States: Saunders Ltd.
Pp. 265–418. Abraini, JH; Gardette-Chauffour, MC; Martinez, E; Rostain, JC; Lemaire, C (1994). Journal of Applied Physiology. American Physiological Society. 76 (3): 1113–8. Retrieved 1 March 2009.
Powell, Mark (2008). Deco for Divers. Southend-on-Sea: Aquapress. P. 70.
Francis, T James R; (2003). '10.6: Manifestations of Decompression Disorders'. In Brubakk, Alf O; Neuman, Tom S (eds.). Bennett and Elliott's physiology and medicine of diving (5th Revised ed.). United States: Saunders Ltd.
Pp. 578–99. Neuman, Tom S (2003). '10.5: Arterial Gas Embolism and Pulmonary Barotrauma'. In Brubakk, Alf O; Neuman, Tom S (eds.). Bennett and Elliott's physiology and medicine of diving (5th ed.).
United States: Saunders Ltd. Pp. 557–8. Neuman, Tom S (2003). '10.5: Arterial Gas Embolism and Pulmonary Barotrauma'. In Brubakk, Alf O; Neuman, Tom S (eds.).
Bennett and Elliott's physiology and medicine of diving (5th ed.). United States: Saunders Ltd.
Pp. 568–71. Bennett, Peter B; Rostain, Jean Claude (2003). '9.2: Inert Gas Narcosis'.
In Brubakk, Alf O; Neuman, Tom S (eds.). Bennett and Elliott's physiology and medicine of diving (5th ed.).
United States: Saunders Ltd. P. 301.
Lippmann, John; (2005). 'Nitrogen narcosis'. Deeper into Diving (2nd ed.). Victoria, Australia: J L Publications. P. 105.; Rostain, Jean Claude (2003). '9.3: The High Pressure Nervous Syndrome'.
In Brubakk, Alf O; Neuman, Tom S (eds.). Bennett and Elliott's physiology and medicine of diving (5th ed.). United States: Saunders Ltd.
Pp. 323–8. Clark, James M; Thom, Stephen R (2003). '9.4: Oxygen under pressure'. In Brubakk, Alf O; Neuman, Tom S (eds.). Bennett and Elliott's physiology and medicine of diving (5th ed.). United States: Saunders Ltd.
Pp. 358–360. Clark, James M; Thom, Stephen R (2003). '9.4: Oxygen under pressure'. In Brubakk, Alf O; Neuman, Tom S (eds.).

Bennett and Elliott's physiology and medicine of diving (5th ed.). United States: Saunders Ltd. P. 376. Donald, Kenneth W (1947). British Medical Journal. 1 (4506): 667–72.Further reading.
(PDF). European Committee for Hyperbaric Medicine. September 2003. Retrieved 22 November 2010.
Donald, Kenneth W (1947). British Medical Journal. 1 (4507): 712–7. Francis T James R, Smith DJ (eds) (1991).
42nd Undersea and Hyperbaric Medical Society Workshop. UHMS Publication Number 79(DECO)5-15-91.
Archived from on 2011-07-27. Retrieved 2011-03-11. CS1 maint: extra text: authors list. Bevan, John (1999). Retrieved 2011-06-13.